Tungsten Countertop
How far would a tungsten countertop descend if I dropped it into the Sun?
Michael Leuchtenberg
Not very far.
With its high melting point, tungsten is a good[1]Except that the word "good" implies that you have some kind of goal you're working toward, which—given how strange the thing you're doing is—I'm not sure about. choice for a sundiving countertop.
The first problem would be sunlight. As the countertop approached the Sun, it would heat up. Tungsten has the highest melting point of any element, but the Sun is one of the meltiest things in the Solar System. When the countertop got within a couple of solar radii, it would liquify.
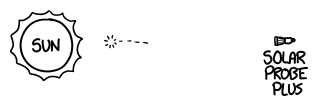
If you protected it with a heat shield of some kind, or dropped it from a sundiving spaceship, it could enter the Sun's atmosphere relatively intact. In this case, some interesting physical effects would destroy it.[2]"Some interesting physical effects would destroy it" summarizes the answers to a large percentage of the questions submitted to this blog.
Because the Sun is so heavy, anything that falls to its surface will be accelerated to a tremendous speed by its gravity. A falling countertop would reach speeds of over 600 km/s—0.2% of the speed of light. (Our rockets can only accelerate spacecraft to 10-20 km/s.)
From the countertop's point of view, particles from the Sun's atmosphere would be slamming into it at 600 km/s. These particles would pack quite a punch.[3]From a particle physics point of view, the individual protons would have energy of about 2 keV. When the countertop got within about a few thousand kilometers[4]I don't want another unit-related word to mentally keep track of, but it's always seemed a little weird that we don't call thousands of kilometers "megameters". of the Sun's surface, these collisions would start delivering more energy to the countertop than the sunlight.
The collisions would fling individual tungsten atoms away from the surface—a process called sputtering. Between the sputtering and ordinary heating from the impacts, the countertop would start to absorb a lot of energy very quickly.
If the countertop were larger, it could penetrate into the Sun's photosphere—the first layer beneath the "surface"—and potentially trigger a solar flare.[5]For a scientific discussion of this, see the article Impacts of comets onto the Sun and coronal mass ejections. For a science fiction discussion of this, see Stephen Baxter and Arthur C. Clarke's 2005 novel Sunstorm. But as it is, it wouldn't even make it through the Sun's atmosphere—it would be vaporized somewhere in the Sun's chromosphere.
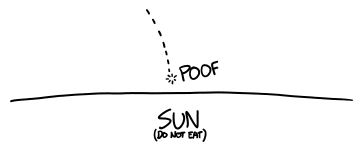
This brings us around to a key question:
Who the hell has tungsten countertops?
Sure, it has good heat tolerance. But I'd be nervous about using tungsten as a food-preparation surface.
In September of 1994, a French soldier drank wine from a rifle barrel. Fifteen minutes later, he started having seizures. He was rushed to the hospital and treated for "acute tungsten intoxication"—the first known case in medical history. He reportedly made a full recovery, although concentrations of tungsten were present in all his body tissues for weeks.
The lesson here is to use normal materials. Michael, just make your countertops out of granite and don't drop them in the Sun.
And if you're a French soldier, please just drink wine like a normal person.
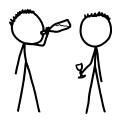